Finally! The last 'What is....?' of the series. Well, don't think we're out of the woods already, there is still a bit more left to learn, as we qnswer the question, 'What is Physics?'
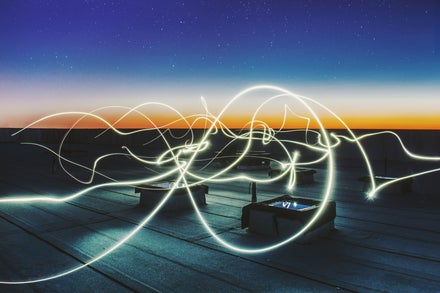
THE ELECTROMAGNETIC SPECTRUM
Electric charges as well as current and magnetism exert influence across space. In 1873 James Clerk Maxwell published his highly significant theory of electromagnetism, which brought the phenomena of electricity, magnetism, and light together in a unified framework. He theorized that electromagnetism moves across space at the speed of light in waves composed of electric and magnetic fields. He also concluded that light is such an electromagnetic wave.
In the 1880s Heinrich Hertz deliberately produced and detected such waves. The waves he produced had wavelengths far longer than those of visible light; they are now known as radio waves. Guglielmo Marconi used this discovery in developing the radio.
Infrared and ultraviolet radiation were found to fit the electromagnetic wave model as well. So too did X-rays, which were discovered by Wilhelm Roentgen in 1895. This seemed to prove that all radiant energy moves in waves. However, another phase of Hertz’s work (and work done by others) revived the particle theory.
THE NATURE OF ELECTRICITY AND THE ATOM
During his experiments, Hertz had found that light falling upon metal would drive out a negative charge. (This phenomenon is the photoelectric effect discussed yesterday.) Further experiments suggested that the charge might consist of particles. Evidence of particles was also found in electric discharges through a vacuum, and in 1896 Henri Becquerel discovered the natural radioactivity of uranium. Shortly after, Marie and Pierre Curie discovered radioactive polonium and radium. Studies revealed that there are three kinds of radioactive rays, called alpha, beta, and gamma. Alpha rays were found to be positively charged particles; beta rays were negatively charged particles; and gamma rays were electromagnetic pulses that carried more energy than X-rays.
Other experimenters proved that electrons exist and that they are one of the building blocks of atoms. Beta rays were proved to be electrons. By 1900 it was clear that atoms are made of smaller electrified particles.
It also became clear that free electrons exert a negative charge (measured by Millikan in 1909) and that electric current consists of electrons moving through a conductor. Thus electricity and atoms are intimately connected, and both are particle in nature.
Since atoms are electrically neutral, they must contain positive and negative charge in equal amounts. Presumably, the negative charge was in the form of electrons. However, questions remained about the nature, location, and distribution of positive charge in atoms.
In 1904 J.J. Thomson proposed his “plum pudding” model, in which the electrons (the “plums”) are embedded in a sort of positive fluid (the “pudding”). In 1911, however, Ernest Rutherford conducted experiments that suggested otherwise. He fired alpha particles at gold foil and found that only a tiny fraction of them bounced back; the rest went right through. This suggested that the deflected alpha particles had interacted with other positively charged particles in the gold foil; the others had not. He interpreted this to mean that the positive charge is contained in a tiny nucleus, with most of the atom being empty space. He thought that the electrons might circle the nucleus, much like planets orbit the Sun.
THE BIRTH OF QUANTUM MECHANICS
As discussed earlier, Planck and Einstein had explained experimental results using the concept that electromagnetic energy interacts with matter in the form of particles—whose energy is connected with the wavelength ascribed to that color of light. In 1913 Niels Bohr considered the problem of how electrons and the positively charged nucleus could coexist in atoms. Like Rutherford, he believed that the electrons orbit the nucleus.
The problem, though, was that Maxwell’s theory predicted that such an orbit would decay, with the electron falling into the nucleus in a short time. To avoid this problem, Bohr postulated that only certain, very specific amounts of orbital energy are possible in an atom. This allows the atom to exist in stable states. If the electron suddenly “falls” from a higher energy to a lower one, it gives off an exact amount of energy in the form of a photon of light, whose wavelength is determined from its energy by the same rule found by Planck and Einstein.
Light, which had seemed earlier to be a wave, now seemed to have definite particle properties. In 1923 Louis de Broglie suggested that particles such as electrons also might travel in the form of waves. This was confirmed by experiment in 1927. Thus it seemed that both the particle theory and the wave theory must be correct, for both light and matter. The problem was to find how both could be true.
By 1927 physicists accomplished this by developing a consistent body of physical laws called quantum mechanics. Quantum mechanics is an outgrowth of Planck’s quantum theory. It is the branch of physics that deals with the behavior of matter and light at atomic and subatomic scales. Among the founders of quantum mechanics were the mathematical physicists Max Born, Werner Heisenberg, de Broglie, Erwin Schrödinger, P.A.M. Dirac, and Pascual Jordan.
Heisenberg stated that it is impossible to measure, simultaneously and exactly, both the position and the velocity of a particle. The more accurately one measures the position, the less accurately one can measure the velocity, and vice versa. Schrödinger developed an equation that describes the wave that governs the motion of the associated particle. According to this equation, the particle is most likely to be found in those places where the undulations of the wave are greatest or most intense. Schrödinger’s wave equation became the fundamental equation of quantum mechanics. The theory does not explain how the wave pattern arises or why it directs the movement of particles but simply describes in mathematical terms how the latter happens.
SPECIALTIES IN PHYSICS
The branch of physics that studies objects on the largest scale is known as astrophysics. Astrophysics is a branch of astronomy that is primarily concerned with the physical properties and structures of cosmic objects.
A branch of physics that studies matter on a scale more familiar to most persons is solid state physics, which explores the internal structure and properties of solids. The triumph of solid state physics in the 20th century was the development of such devices as the transistor, the light-emitting diode (LED), and the photoelectric cell. Solid state physics is part of the field known as condensed matter physics, which also studies liquids.
Another branch of physics that deals with matter in bulk quantities is plasma physics. Plasma is the state of matter found in stars, and plasmas can be hot enough for thermonuclear reactions to take place in them.
Cryogenics is the study of the behavior of matter at very cold temperatures. The properties of many materials alter considerably at such low temperatures. Certain metals and ceramic compounds, for example, lose all resistance to electrical current and become superconducting.
Many other branches of physics also deal with aspects of matter on scales with which humans are familiar. Biophysics studies the physical laws that give rise to or affect biological processes. Acoustics is the study of the behavior of sound, and optics is the study of the behavior of light. It encompasses the general study of force and the motion of objects. Thermodynamics is the study of the relationship between heat, work, temperature, and energy. Physicists also study electromagnetism.
Molecular physics deals with the way the structure of atoms affects their bonds with each other. Atomic physics is the study of the structure and behavior of the atom itself. These studies have contributed to the understanding of nuclear energy levels.
Nuclear physicists study the structure of the atom’s nucleus. They investigate the special forces that act between nuclear particles (the weak and strong interactions) and the radiation from unstable nuclei. They also study the nuclear reactions known as fission, in which nuclei split into smaller parts, and fusion, in which nuclei merge to form larger nuclei. The energy released during these reactions has been put to use in nuclear power plants and in nuclear weapons. Perhaps the most challenging problem concerns how to relate quantum mechanics, which very accurately describes matter on the smallest scales, and general relativity, which beautifully describes space, time, and gravity on larger scales. It is believed that these two rather dissimilar theories could be united by a theory of “quantum gravity.” Various approaches are under investigation. Many physicists are especially hopeful that “string theory” or a deeper “M-theory,” in which space-time has 10 or 11 dimensions, may finally solve this problem—and also provide a fully unified description of all the forces and elementary particles.
So that's it! We've finished. Next, we are going to do a surprise topic. As always, stay curious, and stay sciencey!
Create Your Own Website With Webador