Yesterday, we began to look at what physics is. Today, we are going to carry on with this.
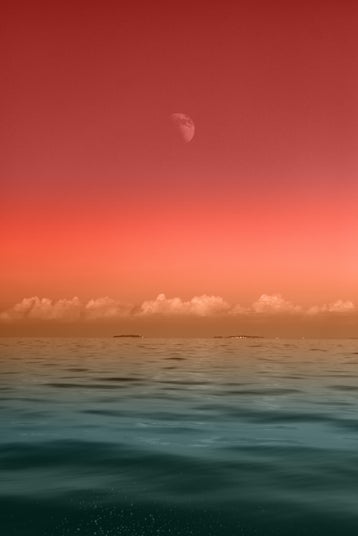
THE SUCCESS OF NEWTONIAN MECHANICS
Scientific reasoning based upon these principles came to be called Newtonian mechanics and (in the 20th century) classical physics. Newtonian mechanics was remarkably successful in accounting for the motions of the Moon, Earth, and other planets. In fact, Newton’s methods proved adequate for explaining all known motions and mechanical effects for some two centuries after his time.
During the 18th century mechanics was developed into a well-integrated and mature science through the work of men such as Leonhard Euler, the Bernoullis, and Joseph-Louis Lagrange. In the 19th century the theory of gyroscopes was developed, and the kinetic theory of heat was worked out in terms consistent with Newtonian mechanics.
By the late 19th century, Newtonian mechanics had triumphed in so many areas that some physicists began to believe that physics itself was nearly complete. Such speculations soon proved premature, however.
RELATIVITY
In 1873 the Scottish physicist James Clerk Maxwell showed that light travels as a wave. This remarkable finding raised an important question: If light is a moving wave, what substance supports it? Ocean waves consist of the vibrations (tiny back-and-forth movements) of molecules of water. Sound waves are formed from the vibrations of molecules of gases in the air. But what vibrates to make a moving light wave? Or to put it another way, how does the energy embodied in light travel from point to point?
Scientists of the time believed that light traveled through an invisible substance called the ether. Supposedly, this ether was spread through all of space but did not interfere with the motion of the planets and stars. Scientists thought that Earth moved (“drifted”) through the ether. In 1887 the American physicists A.A. Michelson and E.W. Morley tried to measure this drift. Although they made extremely precise measurements, they detected no sign of Earth’s motion. This surprising result meant that there was no such thing as ether. It also showed that Newtonian mechanics could not explain the behavior of light.
Michelson and Morley had measured the speed of light accurately as it traveled both in the same direction as Earth’s movement and in the direction opposite to Earth’s movement. They expected to get slightly different values. They believed that Earth’s speed would be added to or subtracted from the speed of light. This is similar to a situation in which a passenger in a train moving at 100 miles per hour shoots an arrow in the train’s direction of motion at 200 miles per hour. An observer standing by the tracks would measure the speed of the arrow as the sum of the two speeds, or 300 miles per hour.
Michelson and Morley discovered, however, that light does not behave that way. The speed of light is about 186,300 miles (299,800 kilometers) per second. Evidently, the speed of light plus any other added speed was still equal only to the speed of light. To explain the result of the Michelson-Morley experiment, physics had to be recast on a new foundation.
After physicists had struggled for years with the problem, Albert Einstein in 1905 provided a solution with his first (or special) theory of relativity. He took as a working principle that the speed of light is always the same for all observers, no matter how fast they are moving. He also said that all observers moving at constant speeds in a straight line should be able to discover the same laws of physics, whether in the realm of mechanics, or of electricity, magnetism, and light.
Through mathematical equations, Einstein showed that, if these two principles were true, Newton’s concepts of space and time could not be correct. In Newtonian mechanics, space and time are “absolute.” In other words, it is assumed that all observers everywhere in the universe will obtain identical measurements of space and time. According to special relativity, the speed of light is constant, but observers in motion relative to one another will not obtain the same measurements of space and time. Einstein also considered space and time to be aspects of a single entity, called “space-time.” He further determined that light travels at the fastest possible speed in the universe; material objects can approach but never reach its speed.
Special relativity draws many startling conclusions that are contrary to everyday experience. For instance, it concludes that as a body moves faster, it becomes smaller along its direction of motion. Time also runs more slowly for the body than it does for something that is not moving as fast. At the speeds of everyday life, these differences are so tiny as to be unnoticeable. At lower speeds the predictions of relativity thus match Newton’s beautifully. At extremely large speeds, the differences in measurements are much greater. For example, suppose that one of two identical twins flies off to a distant star at nearly the speed of light. When she returns to Earth, she finds that she is much younger than the twin she had left behind. Because the first twin had been traveling in a spacecraft at an extremely fast speed, time passed more slowly for her. As strange as such results are, numerous experiments have shown special relativity to be true.
In 1916 Einstein published his second (or general) theory of relativity, which concerns gravity. As in special relativity, the predictions match those of Newton quite closely in ordinary circumstances, but they depart significantly in cases in which gravity is very strong.
Whereas Newton thought that gravity was a force, Einstein showed that gravity arises from the shape of space-time. Each mass in the universe bends the very structure of space-time around it. This concept is difficult to visualize, but an analogy can provide some insight. Consider space-time to be a rubber sheet spread out flat. Imagine that a bowling ball (representing the Sun) is placed on the sheet. The ball will curve the sheet (space-time) around it, creating a cuplike depression. Next, imagine that a marble is also put on the sheet. A smaller depression will form around the marble. If the marble is placed near the bowling ball’s depression, it will roll down the slope toward the ball as if pulled by a force. If the marble is given a sideways push, it will travel around the bowling ball like a planet orbits the Sun. It is as if a steady pull toward the ball swings the marble into a closed path.
Both of Einstein’s relativity theories have so far proved correct in every case in which it has been possible to test them. Today relativistic corrections to Newtonian mechanics are made whenever needed. These theories, together with other 20th-century developments, have been particularly helpful in working out the modern theory of the atom and are also the basis for models of the entire universe.
OLD AND NEW CONCEPTS OF HEAT
Physicists now regard heat as being essentially the random energy of motion of the tiny parts (molecules) of which most matter consists. This theory, called the kinetic molecular theory, was developed largely in the 19th century. It was not established, however, until after centuries of working with mistaken concepts.
The ancients thought that heat was an element. Empedocles proposed that the roots of all things are the four elements—fire (heat), air, water, and earth. Therefore heat could only be analyzed as a physical entity that flows into and out of substances. This idea endured for centuries. Even as brilliant a scientist as Antoine-Laurent Lavoisier, toward the end of the 18th century, considered heat as a fluid of some sort, which he called caloric.
Experiments constantly disproved this view. Weighing matter when it was hot, then cold, showed no change that would correspond to a flow of caloric in or out of the substance. In the late 18th and early 19th century a group of physicists, including Benjamin Thompson, developed a new theory of heat. They reasoned that motion is transformed into heat (as when a bullet strikes through a piece of wood). Thus they concluded that heat is not an element or a substance. It is a result rather of the constant motion of the invisible particles that compose matter, which we now call molecules. Many different experiments confirmed this and thus established the kinetic molecular theory.
This theory proved satisfactory for explaining the heat energy contained in matter. At the same time physicists developed another theory to explain radiant heat, or heat transmitted through radiation such as light, rather than through matter. They concluded that radiant heat passing through empty space in the form of light is a type of wave, in a hypothetical substance called “ether” (whose existence was eventually disproved, as mentioned earlier). The waves are emitted by a hot body such as the Sun. When they strike matter (as on Earth), they stimulate the molecules in the matter to greater motion. This “heats” the matter.
PLANCK’S QUANTUM THEORY OF HEAT
According to this theory, heat can be emitted and absorbed in any amount (and thus can be divided into infinitely smaller amounts). In 1900, however, Max Planck forced a change in this view. He had experimented with a a device known as a blackbody radiator. This hollow object absorbs heat energy sent into it through a hole, then reradiates the energy somewhat as iron would. As iron is heated more and more, it first gets hot, then glows with dull red light, and finally becomes white hot—meaning that it is emitting every wavelength of light in the spectrum, including radiant heat (infrared radiation) and ultraviolet radiation.
If heat energy could indeed be divided into smaller and smaller amounts, the energy emitted in these experiments would have a particular distribution of wavelengths. But it did not. Earlier attempts to explain the form of the spectrum of blackbody radiation had failed badly, predicting far too much radiation at short wavelengths. Planck found a rather different—and very successful—explanation for the observed spectrum, however. He proposed that radiant heat energy is not infinitely divisible. Instead, it is transferred in exact amounts, more like particles than like waves. There is thus a certain smallest particle that cannot be divided. Planck called this smallest particle a quantum.
Since Planck’s experiments were unquestionably accurate, physicists had to accept this quantum theory. Soon the same sort of indivisible unit was found in light and in electricity, and today the quantum theory ranks with relativity as one of the cornerstones of modern physics. The only change from Planck’s view is a later theory that says that quanta travel in association with waves, as explained later in this blog.
ACOUSTICS: THE SCIENCE OF SOUND
Much of what is known about the world was learned through sight and hearing. The ancients naturally were interested in light and sound. Of the two, sound was much easier to understand, and people began discovering facts about sound at an early date.
The physics of sound is called acoustics. Humans’ love of music led them to build musical instruments from which they learned that all bodies that produce sounds are vibrating. Faster vibrations produce sound of higher pitch; and slower vibrations, lower pitch. The ancient Greek mathematician Pythagoras was the founder of acoustics. He investigated the laws of stringed instruments in the 6th century bc. Some 500 years later Vitruvius, a Roman architectural engineer, wrote that sound is produced in the form of concentric waves like those made by a stone when it is thrown into still water. Today it is known that sound waves consist of an ever-widening sphere of compressions, regions where the air molecules are crowded together. These compressions are separated by rarefactions, regions where the molecules are farther apart than usual.
The first known attempts to measure the speed of sound came in the early 17th century. Later Newton developed a theory that predicts the speed of sound from the elastic properties of the air, according to Newtonian mechanical principles.
THE PROBLEM OF EXPLAINING LIGHT
The ancients were familiar with many optical phenomena such as shadows, rainbows, and the use of fire in lamps to produce light. They knew about reflection and that light bends when it goes from water to air (refraction). Lenses were made and used.
In 1608 Hans Lippershey built what may have been the first telescope. Shortly after, Galileo made a telescope with which he discovered Jupiter’s major satellites, the crescent phases of Venus, sunspots, and the rotation of the Sun. At about this same time the microscope came into use. With these optical instruments, great strides were made in astronomy and in the biological sciences.
In the mid-17th century two great physicists, Newton and Christiaan Huygens, performed experiments with light and arrived at two very different conclusions. Newton accepted the theory that light rays are streams of tiny particles. He explained that light bends as it goes from air to water because the particles are attracted toward and pulled downward into the water, gaining speed as they enter the water. Huygens developed a wave theory of light. According to his wave theory, light bends when it goes from air to water because the waves move more slowly in water than in air.
For more than 100 years the argument raged between supporters of the particle and wave theories. At length certain experiments gave support to the wave theory. When two beams of light overlap at a point, they may add to each other to produce brighter light, or they may cancel one another out and produce darkness. This phenomenon is called interference, and it is a property of waves. Shortly after 1800, Thomas Young and later Augustin-Jean Fresnel performed many experiments that demonstrated the interference of light. Their results supported the wave theory. In about 1850 François Arago, Jean-Bernard-Léon Foucault, and Armand-Hippolyte-Louis Fizeau showed that light travels faster in air than in water—just as Huygens had concluded. This was a great triumph for the wave theory, and it convinced physicists that it was the correct explanation.
Then in 1900 it became evident that sometimes light shows particle properties. For example, in the photoelectric effect, light falling on metal is found to eject electrons from the surface in a way for which the wave theory is unable to account. Einstein solved this problem in 1905. He proposed that light interacts with the surface of the metal as a particle (called a photon). However, the energy of the particle is directly proportional to the frequency of its wavelike vibrations—which is what Planck had assumed to explain blackbody radiation. It turns out that, in some sense, Newton and Huygens were both right, but in a way that neither would have expected.
READING THE RIDDLES OF ELECTRICITY
Even before the particle properties of light were understood, physicists had shown that light is the result of electrical activity in atoms. Knowledge of electricity is thus necessary to understand the behavior and properties of light. Lightning, electric eels, and magnetic stones had been known since ancient times. The ancients also knew that if one rubbed a piece of amber with fur it would attract bits of straw. Nevertheless, knowledge of electricity developed much more slowly than any other branch of classical physics.
In the 17th and 18th centuries this previously neglected subject received much attention. It was shown that many materials could be “electrified” by rubbing them with silk or fur. Such experiments seemed to indicate that two kinds of electricity (called charges) might exist. Each seemed to repel others of the same kind and attract their opposites. This could happen only if there were two kinds of charges or if one kind existed in excess and deficiency—that is, in positive (+) and negative (–) amounts. An excess would tend to flow toward (be attracted by) a deficiency. This latter view—which turned out to be correct—was favored by Benjamin Franklin, among others.
During this period, experimenters were hampered by the fact that whenever a path was formed between opposite charges, the charges seemed to unite almost instantly. Then in 1800 Alessandro Volta announced the discovery of an electric battery that would produce a steady flow of direct electric current. This stimulated research in electricity to an unprecedented degree. Humphry Davy, Hans Christian Ørsted, and Michael Faraday used this new source of electricity to discover many new facts. They found, for example, that an electric current can be used to break up dissolved substances, in a process called electrolysis. They also discovered that an electric current can generate magnetism and vice versa.
That's it for today! Tomorrow, we will finish up this topic. Until next time, stay curious, and stay sciencey!
Create Your Own Website With Webador