Today, we are going to look at the last part of this series- what Chemistry is, and this will lead onto what physics is.
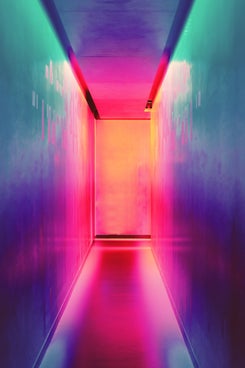
HISTORY OF CHEMISTRY
Modern chemistry is only about two centuries old. The earlier history of chemistry may be divided into three periods: magic; alchemy; and “primitive modern,” a period of transition between alchemy and truly modern chemistry.
THE PERIOD OF MAGIC
The period of magic extended from prehistoric times to about the beginning of the 1st century ad. Most people believed that natural processes were controlled by spirits, and they relied on magic to persuade the spirits to help while they conducted practical operations. Very little progress was made toward understanding how the universe is made, but much practical knowledge was gathered. Perhaps 9,000 years ago, people devised reliable techniques for making and sustaining fire. Gradually they learned to use fire to harden pottery, extract metals from ores, make alloys, and develop materials such as glass. Certain elements that occur naturally in a pure state, such as gold, copper, and sulfur, were recognized and valued for their properties. This was the period of the Sumerian, Babylonian, Egyptian, and Greek cultures.
About 400 bc the Greek philosopher Democritus theorized that all matter was made up of tiny, indivisible units he called atoms, but his idea was not based on scientific evidence. Other Greek philosophers, including Thales and Aristotle, also speculated on the nature of matter, though their theories, too, had little in common with modern chemical knowledge. They believed that earth, air, fire, and water (some imagined a fifth substance called “quintessence”) were the basic elements of all matter. They speculated on the possibility of removing such qualities as hardness, heat or cold, and color from common materials and combining them to make rarer or more valuable substances. They knew that iron could be drawn from a dirty, brown earthen rock and that bronze was made by combining copper and tin. Therefore it seemed possible that if yellowness, hardness, and other qualities could be properly combined, the product would be gold. Such speculations gave rise to alchemy.
THE STERILE PERIOD OF ALCHEMY
The span of time from about the beginning of the 1st century ad to about the 17th century is considered the period of alchemy. The alchemists believed that metals could be converted into gold with the aid of a marvelous mineral called the philosopher’s stone, which they never succeeded in finding or making. They did discover new elements, and they invented basic laboratory equipment and techniques that are still used by chemists. However, the alchemists learned very little that was worthwhile concerning the fundamental nature of matter or of chemical behavior. They failed because their basic theories had almost nothing to do with what actually happens in chemical reactions.
In the 13th century such men as Roger Bacon, Albertus Magnus, and Raymond Lully began to realize how futile it was to search for the philosopher’s stone. They suggested that alchemists might rather seek to help the world with useful new products and methods.
In the 16th century, another important leader in the new trend was Theophrastus Bombastus von Hohenheim, an aggressive, talented Swiss who used the Latin name Paracelsus. He insisted that the object of alchemy should be the cure of the sick. The elements, he said, were salt, sulfur, and mercury (long connected with the “elixir of life,” another nonexistent alchemical substance), and they would give health if present in the body in the proper proportions. On this basis he practiced medicine and attracted many followers. Thus began iatrochemistry, or chemistry applied to the study of medicine and the treatment of disease.
One of the first scientific chemists was Robert Boyle. In 1660 he helped found one of the first scientific organizations in Europe, the Royal Society of London. In a book called The Sceptical Chymist (1661) he rejected previous theories of the composition of matter and compiled the first list of the elements that are recognized today. He also discovered the relationship between the volume and the pressure of a gas.
THE BEGINNINGS OF MODERN CHEMISTRY
For about two centuries after Boyle, scientists continued to make useful discoveries but made little progress in understanding the true nature of matter or chemical behavior. Perhaps the greatest source of confusion and defeat in these centuries was a theory of burning (combustion) called the phlogiston theory. It was originated by the German chemists Johann Joachim Becher and Georg Ernst Stahl in the late 1600s. According to this theory, phlogiston, an “essence” like yellowness or hardness in the theories of the ancient philosophers, escaped from substances during the burning process. By this time, chemists were learning to gain knowledge the modern way: by testing theories with experiments. But such tests failed to confirm the existence of phlogiston.
The first clue to a more useful theory came when an English chemist, Joseph Priestley, discovered in 1774 that a gas (now known as oxygen) was essential to the burning process. (Oxygen was also discovered by the Swedish chemist Carl Wilhelm Scheele at about the same time.) A few years earlier another English scientist, Henry Cavendish, had identified hydrogen as an element. The French chemist Antoine-Laurent Lavoisier used the discoveries of Priestley and Cavendish in a series of experiments from which he formulated the presently accepted theory of combustion. He also showed that burning, the rusting of metals, and the breathing of animals are all processes in which oxygen combines chemically with other substances. Lavoisier’s most significant finding was that the products of a chemical reaction have the same total mass as the reactants, no matter how much the substances are changed. This means that, even when chemical changes take place, something essential stays the same. These contributions are often considered to mark the beginning of modern chemistry.
DALTON’S THEORY OF ATOMS
Lavoisier’s results gave chemists their first sound understanding concerning the nature of chemical reactions. The next milestone was the atomic theory, advanced in 1805 by an English schoolteacher, John Dalton. This theory states that matter is made up of small particles called atoms, that each chemical element has its own kind of atoms (in contrast to earlier ideas that atoms are essentially alike), and that chemical changes take place between atoms or groups of atoms. To support his theory Dalton set about calculating the relative weights of the atoms of several elements. The Swedish chemist Jöns Jacob Berzelius greatly expanded this work in a long series of experiments in which he found accurate atomic weights for about 40 elements. He also found chemical formulas for most of the inorganic compounds known at that time.
Equipped at last with sound views about the nature of matter and of chemical reactions, chemistry made rapid advances. About 1811 came the hypothesis of Amedeo Avogadro, an Italian chemist, about the number of molecules in a given volume of gas. To Dalton’s theory that the atoms of a single element all have the same weight, Avogadro added the following notions: that equal volumes of different gases at the same temperature and pressure contain the same number of molecules, and that some of the gaseous elements are found in two-atom molecules rather than as independent atoms. These theories explained why only half a volume of oxygen was needed to combine with a volume of carbon monoxide (CO) to form carbon dioxide (CO2). Oxygen gas is made of two-atom molecules, and one oxygen molecule reacts with two molecules of carbon monoxide: O2 + 2CO→2CO2.
THE RISE OF ORGANIC CHEMISTRY
The vast new field of organic chemistry was opened in 1828 with Friedrich Wöhler’s synthesis of urea, a compound present in certain body fluids of mammals, from inorganic materials in his laboratory. This disproved the assumption that such compounds could be formed only through the operation of a “life force” present in animals and plants. About this time chemists also began to realize that a molecule’s geometric structure had a great effect on its chemical and biological properties. This applied especially to the structure of organic compounds, in which carbon atoms provide the basic framework. Chemists found, for example, that two organic compounds can have the same kinds and numbers of atoms but different properties because their molecules are put together differently. Such compounds are called isomers. The French chemist Louis Pasteur opened the way for the study of carbon’s special three-dimensional bonding geometry in his investigation of the isomers of tartaric acid, an organic compound found in grapes.
The German chemist Friedrich Kekulé showed in 1858 that carbon atoms can combine with four other atoms and link with each other to form long chains. He also proposed the cyclic (ring) structure of benzene about this time. Another important field, electrochemistry, was born in the 1830s when Michael Faraday formulated its laws.
MORE NEW FIELDS OF INVESTIGATION
By the middle of the 19th century, about 60 elements were known. A few chemists had noticed that certain elements were much alike in their properties and saw a pattern emerge when elements were arranged according to atomic weight. The work of these men enabled the Russian chemist Dmitri Mendeleev, in 1869, to publish the first periodic table. Mendeleev predicted correctly that the gaps left in his table would be filled by as yet undiscovered elements with properties that he also predicted. This table became the foundation of theoretical chemistry.
To this period belongs Robert Bunsen, whose most important contribution to chemistry was the organization of the field of spectroscopy, based on observations that each element when heated emits light having a characteristic color, or wavelength. To aid his research, he invented many instruments, including the spectroscope and the Bunsen burner.
In the second half of the 19th century, Pasteur, who had done his research on isomers as a young man, became interested in how yeast and bacteria are involved in chemical reactions and in causing illness. He invented the process of pasteurization to prevent foods and beverages from spoiling, and he proved that microorganisms are responsible for the chemical changes called fermentation in beer and wine and for several diseases of humans and other animals. He was also the first to use vaccines against anthrax, chicken cholera, and rabies. His achievements helped lay the groundwork for both biochemistry and microbiology.
THE DEVELOPMENT OF PHYSICAL CHEMISTRY
The area where physics and chemistry overlap, which had been explored by Avogadro in his investigations of gas volumes, became prominent in the last quarter of the 19th century. Wilhelm Ostwald and Jacobus van’t Hoff used physical principles to describe the energy changes accompanying reactions in solution that go on simultaneously in opposite directions (reactants to products and products to reactants). Svante August Arrhenius proposed the idea that compounds such as acids and bases form ions (electrically charged particles) when they dissolve in water, and that these ions allow the solutions to conduct electricity.
The American chemist Willard Gibbs developed the “phase rule,” a mathematical formula showing how temperature and pressure affect the capacity of substances to exist in equilibrium in as many as three different states of matter, or phases (solid, liquid, and gas). Accurate atomic weight determinations were the work of Theodore Richards. Between 1894 and 1898, the efforts of the chemist William Ramsay and the physicist Lord Rayleigh (John William Strutt) resulted in the isolation of helium on Earth (the element previously had been detected in the sun) and the discovery of the other noble gases.
After 1900, chemists began receiving invaluable aid from discoveries made in physics about the electrical nature of the atom. Henry Moseley, working with X-rays emitted from atoms of the different elements, reorganized the elements using atomic number—a quantity equal to the positive charge of the atomic nucleus—rather than atomic weight. Max von Laue and Sir William Bragg and his son Lawrence Bragg laid the basis for determining the atomic structure of substances in crystal form by means of X-rays. Francis W. Aston developed the mass spectrograph, a device that separates atoms or molecular fragments of different mass, and used it to discover isotopes of many elements. Later Harold C. Urey isolated deuterium, an isotope of hydrogen with one neutron. Deuterium has become important as a chemical tracer, in thermonuclear weapons, and in fusion power research.
NUCLEAR CHEMISTRY AND ATOMIC STRUCTURE
In 1896 Henri Becquerel with Marie Curie and Pierre Curie discovered the phenomenon of radioactivity. Thus, other scientists were shown that atoms were not permanent and changeless, and the basis was laid for nuclear chemistry and nuclear physics. Having found that atoms sometimes transmuted into other elements on their own, scientists attempted to do the same in the laboratory. In 1919 Ernest Rutherford became the first to succeed, using natural radioactivity to transmute nitrogen atoms into atoms of oxygen and hydrogen. In 1934 Frédéric and Irène Joliot-Curie made radioactive isotopes of elements that were not normally radioactive. Five years later Otto Hahn, Fritz Strassmann, and Lise Meitner discovered that the uranium nucleus could be made to fission, or split into the nuclei of lighter elements, by bombarding it with uncharged particles called neutrons.
By the early 1940s nuclear reactions had been used to make radioactive isotopes of all elements; Glenn Seaborg contributed much to this work. In the 1940s and ’50s Seaborg and his co-workers also made several elements not known to exist in nature. The new elements had atomic numbers greater than 92, the atomic number of uranium. By the early 21st century nuclear scientists were adding new elements to the periodic tale with atomic numbers higher than 110.
Rutherford’s discovery in 1911 that the atom has a tiny massive nucleus at its center allowed chemists and physicists such as Gilbert Lewis, Irving Langmuir, and Niels Bohr over the next 20 years to explain chemical bonding and atomic structure in terms of the behavior of electrons orbiting the nucleus. In the late 1920s and early 1930s, Linus Pauling contributed much to knowledge of the nature of the chemical bond and of the relationship between the structure of atoms and molecules and their properties.
NEW SYNTHETIC MATERIALS
Some of the most notable achievements in modern chemistry have come from efforts to create whole new classes of materials. Early plastics such as celluloid, invented in the late 1860s, relied on large molecules found in natural substances. In 1909, however, the Belgian-born inventor Leo H. Baekeland took out a United States patent for a hard, chemically resistant, electrically nonconductive plastic that he called Bakelite. Made from the chemical combination of synthetic compounds called formaldehydes and phenols, Bakelite proved to be exceptionally useful as an electrical insulator and as a structural material for such consumer goods as radio cabinets, telephone housings, and even jewelry.
The commercial success of Bakelite sparked great interest and investment in the plastics industry, in the study of coal-tar products and other organic compounds, and in the theoretical understanding of complex molecules. These research activities led not only to new dyes, drugs, and detergents but also to the successful manipulation of molecules to produce dozens of materials with particular qualities such as hardness, flexibility, or transparency.
Techniques were developed, often requiring catalysts and elaborate equipment, to make these polymers—that is, complex molecules built up from simpler structures. From the field of polymer chemistry, the synthetic rubber and synthetic fibre industries have grown. Synthetic fibres are used in fabrics, carpets, rope, and brush bristles and for producing synthetic rubber.
Another dramatic result of the growth in chemical knowledge has been the expansion of the modern pharmaceutical industry. Notable early achievements include the development of the synthetic drugs acetylsalicylic acid (aspirin) in 1897, Salvarsan (for treating the bacterial disease syphilis) in 1910, and Prontosil (the first sulfa drug for treating bacterial infections) in 1932, as well as the discovery of the antibiotic penicillin (produced naturally by a mold) in 1928.
Since the late 20th century the rapid growth in the understanding of chemical processes in general, and of organic and biochemical reactions in particular, has revolutionized the treatment of disease. Most drugs available today do not occur naturally but are made in the laboratory from elements and inorganic and organic compounds. Others are derived from animals, plants, microorganisms, and minerals, by pharmaceutical researchers who often use chemical reactions to modify molecular structures in order to make drugs that are more effective and have fewer harmful side effects.
THE MERGING OF CHEMISTRY AND OTHER DISCIPLINES
Technological advances in chemistry, physics, and biology in the past few decades have resulted in the breakdown of distinctions between these formerly separate disciplines. Biochemists and biophysicists, for example, have made great advances in understanding photosynthesis, the chemical process by which plants use the energy in sunlight to make food, and in unraveling the role of the complex molecules DNA and RNA in heredity and the synthesis of proteins. Biochemists and molecular biologists have also learned how to transfer DNA from one kind of organism into another kind and modify the code that it carries for guiding the synthesis of proteins. Microorganisms, plants, and animals that have been given segments of human DNA are employed as living “drug factories” to make large quantities of valuable human proteins such as insulin. Researchers are also investigating how to insert DNA molecules into human cells or to chemically modify the DNA present in order to cure diseases in people who have missing or defective DNA.
SOME CHEMICAL TERMS
- atom. The smallest part of an element that retains the element’s properties. An atom cannot be broken down by ordinary chemical means.
- chemical bond. Any of the forces involving electrons that hold atoms together in molecules, crystals, and other stable forms of matter.
- compound. A substance containing two or more kinds of atoms in chemical combination. The atoms in a compound can be separated by chemical means.
- Debye-Hückel equation. A mathematical expression derived to elucidate certain properties of solutions of electrolytes, that is, substances present in the solutions in the form of charged particles (ions). Such solutions often behave as if the number of dissolved particles were greater or less than the number actually present; the Debye-Hückel equation takes into account the interactions between the various ions, which are the principal cause of the discrepancies between the properties of dilute solutions of electrolytes and those of so-called ideal solutions.
- element. A substance composed entirely of atoms of one kind. It cannot be broken down chemically.
- Henry’s law. A statement that the weight of a gas dissolved by a liquid is proportional to the pressure of the gas upon the liquid. The law, which was first formulated in 1803 by the English physician and chemist William Henry, holds only for dilute solutions and low gas pressures.
- mass. The quantity of matter in an object or body.
- matter. Anything that has mass and takes up space.
- mixture. A substance composed of two or more elements or compounds, each of which retains its individual properties.
- molecule. The smallest part of a compound that retains that compound’s properties. Also, two or more atoms of the same element that are chemically combined. The atoms in a molecule can be separated by chemical means.
- physical change. A change in form (solid, liquid, gas, or plasma) but not in chemical composition.
- properties. The characteristics of a substance. The physical properties (mass, melting point, hardness, etc.) can be measured and expressed in numbers. The chemical properties (valence, ionization, etc.) determine how a substance reacts chemically.
- reaction (or chemical change). A change in the chemical composition of a substance.
And that's it! We have finished the chemistry. After 3 parts of blogs, it is finished. Stay tuned, because we will have another parter on what physics is. If you missed it, the links to parts 1 and 2 are below- just press the buttons. As always, until next time, stay curious, and stay sciencey!
Create Your Own Website With Webador